Advanced Culture: Making More ‘Life-Like’ Cellular Models
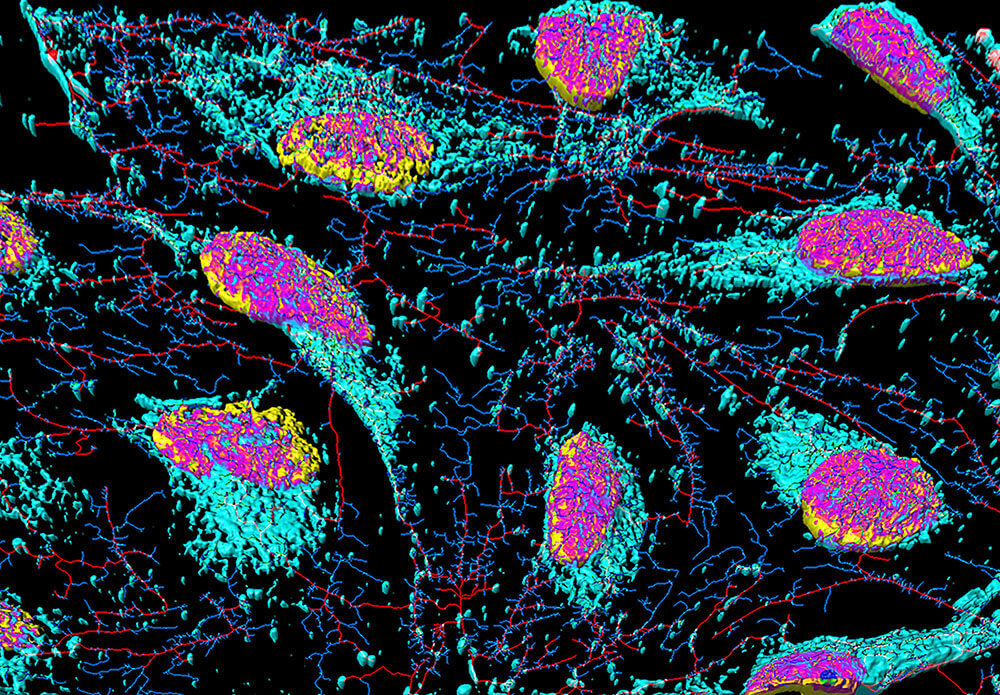
Cellular models, an essential part of developing new medicines, are becoming more realistic and relevant to patients.
Long before a drug candidate enters clinical trials to be tested in humans, it goes through countless rounds of testing over several years in cellular models, also known as cell-based assays. From the earliest stage of identifying active molecules that can interact with a disease target to the later stages of testing toxicity and dosing, cells, particularly human cells, are indispensable to developing new medicines.
Human donor cells are the best model for testing how the body will respond to potential drugs, but they’re also a precious commodity in scientific research. It’s difficult to get a volunteer to donate more than once and samples from brain tissue and other difficult-to-access organs are hard to come by.
For this reason, for decades, researchers have relied mainly on what are known as “immortalized cell lines” to produce the human proteins — the disease targets — to test molecules against. Normal human cells are limited in the number of times they can replicate before they die, but immortalized cells have a mutation that allows them to grow indefinitely, making them dependable workhorses to produce proteins and other biological substances. Among the oldest and most commonly used cell lines in scientific research are HeLa cells, originally taken from a cervical-cancer biopsy in 1951 from Henrietta Lacks, whose cancerous cells showed an amazing ability to divide indefinitely.
Cell Culture Challenges
The challenge with relying on immortalized cell lines is that while the proteins they produce are a close surrogate to those involved in specific model diseases, HeLa cells can’t replicate the full conditions of the native cell biology. For example, researchers can engineer HeLa cells to overexpress a brain protein involved in Alzheimer’s disease, but this model is just not the same as real-life conditions.
“There are other proteins found in brain neurons in addition to the ones that we think might be targets for therapies. You’re not going to have those other proteins in HeLa cells, so they can never truly reflect the full disease state,” says Marie-Claire Peakman, Executive Director, Head Hit Discovery & Lead Profiling, Medicine Design at Pfizer’s Groton, Connecticut, location. “Other proteins in patient brain cells could be interacting with the target protein, such as cofactors and modulators, that are just not there in our model.”
More Life-like Models
Thanks to recent advances in cell culturing techniques, Marie-Claire’s group is now working with much more realistic cellular models earlier on in the drug discovery process. “We really need to start early in the compound screening process to use cells that contain all of the different proteins that are actually expressed in patients. So, when we find a compound that is active in these situations, it’s much more likely to be active when we put it into patients later on in the clinic,” says Peakman. “These advances have the potential to dramatically improve our ability to translate our medicines from early screening actives to effective medicine in patients,” she adds.
Read on to learn more about these advances:
Precious Primary Cells
To start, scientists are employing new cell culturing techniques that are allowing researchers to make better use of the human donor cells they do have. In patient blood samples used to study autoimmune diseases, scientists have found new ways to stimulate certain populations of white blood cells so that they can expand by 100-fold. Some projects can require many billions of cells, according to Peakman, and the ability to expand these donor cell samples allows us to conduct more experiments to be confident in our findings.
Turning Back the Clock
In a case of real-life “Benjamin Button,” scientists now have the ability to take a patient’s skin cells and genetically reprogram them to revert back to immature cells that have not yet differentiated into their specialty function. Known as pluripotent stem cells, these “blank slate” cells can be induced to become whichever specialty cells are needed for experiments, such as neurons or cardiac muscle cells. The advantage to using these induced pluripotent stem (iPS) cells for testing is that they more closely resemble the conditions of the actual patient’s diseased cells.
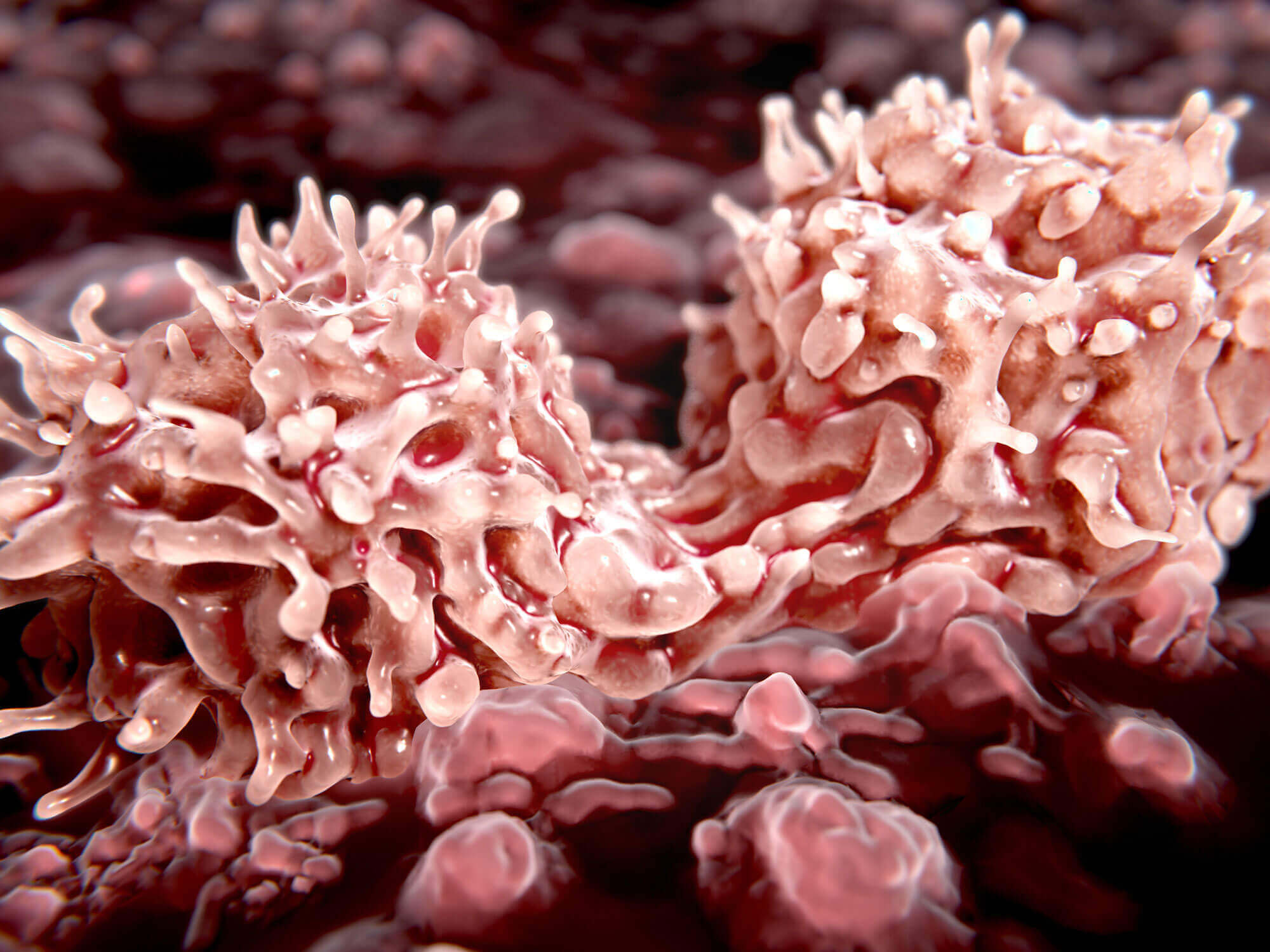
Stem cells are undifferentiated cells that can specialize through mitosis to many other cell types of multicellular organisms. (Getty free)
“The value is that not only are they now expressing all the proteins that the primary cells express, but also the genetic background of the patient who has the disease,” says Peakman. “If it’s a very complex disease with multiple genes or lifestyle factors, we now have cells with all that experience in them.”
The iPS cells, however, are still not considered to be exact matches to the actual target cells in a patient. “The scientific community is still trying to understand how representative these cells are of a patient’s sample,” says Peakman. “And some are better than others. With some cell types, such a hepatocytes (liver cells), we’re not sure we can make them mature enough to express the enzymes needed to test drug metabolism.”
Moving to 3-D
Scientists have also developed techniques to grow cells in 3-D cultures systems, allowing cells to grow in all directions (as opposed to on a flat plate), which more closely simulates the conditions of a living organism. Like a pilot training in a flight simulator, these cell cultures are also exposed to more realistic life-like conditions, such as having bodily fluids flow over them as they grow.
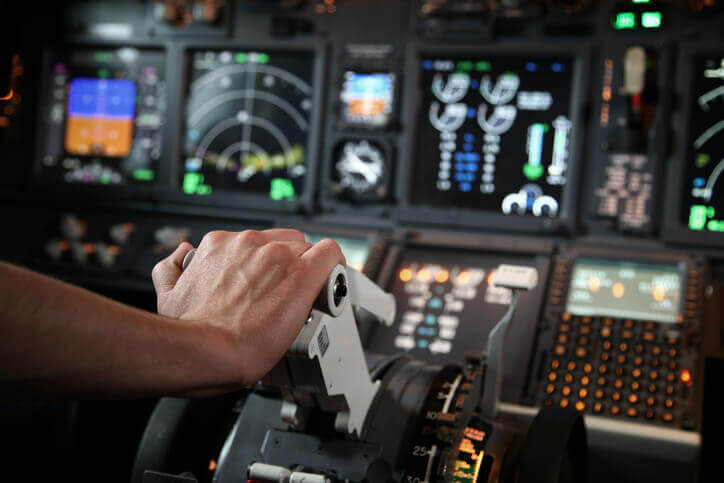
Like a pilot training in a flight simulator, 3-D cell cultures are also exposed to more realistic life-like conditions, such as having bodily fluids flow over them as they grow.(Getty free)
“The shear force of these liquids may induce more expression of a particular protein as happens in the human body,” says Peakman.
Lab-grown models of miniature organs, known as organoids, are also proving important tools to test drug candidates in a more realistic system. Scientists, for example, have created a gut organoid that is modeled after the gut barrier system, a single layer of cells in our intestinal wall that absorbs nutrients and keeps out toxins and dangerous microbes.
“In people with Crohn’s disease and other inflammatory bowel diseases, the gut barrier is compromised. We can better understand these diseases by creating a more physiologically relevant model,” says Peakman.
And these models are not just at the cellular level, read on here to learn how scientists are creating “organ-on-a-chip” models.